In the world of biomedical research, the quest for alternatives to traditional animal testing has led to groundbreaking innovations. One such advancement, organs-on-a-chip, promises to revolutionize how we study human physiology while addressing ethical concerns. This blog post explores the emergence of organs-on-a-chip technology, its ethical implications, advantages over animal testing, and its potential to reshape the future of scientific experimentation.
Introduction
Animal testing has been a critical but controversial method in biomedical research, raising ethical concerns about animal welfare and questions about its relevance in accurately predicting human responses.
These challenges have spurred the development of organs-on-a-chip—a cutting-edge technology that recreates human organ functions within microfluidic devices. By providing a more humane and scientifically robust alternative, organs-on-a-chip promise to revolutionize how we conduct research, offering insights into human biology with greater precision and ethical consideration. The evolution of this technology, its ethical implications, and its potential to reshape the future of scientific experimentation will be discussed in the following.
What are Animal Testing Alternatives?
A wide range of species are used in laboratories worldwide, including mice, rats, rabbits, guinea pigs, hamsters, birds, fish, cats, dogs, farm animals and non-human primates. For instance, it is estimated that more than 115 million animals are used in laboratory experiments globally each year.
What is Animal Testing?
Animal testing refers to the practice of using live animals in experiments to study basic biology and diseases, assess the safety and effectiveness of new medicinal products, and test the health and environmental impacts of consumer and industrial products. These procedures can range from mild to severe, often causing significant physical and psychological distress. Common practices include forced chemical exposure, genetic manipulation, surgical procedures, and various forms of restraint. Unfortunately, most animals used in these experiments are euthanized at the study’s conclusion, while others may be reused in future tests.
Despite some progress, millions of animals still endure the stress and deprivation of laboratory life, often without even being included in official records.
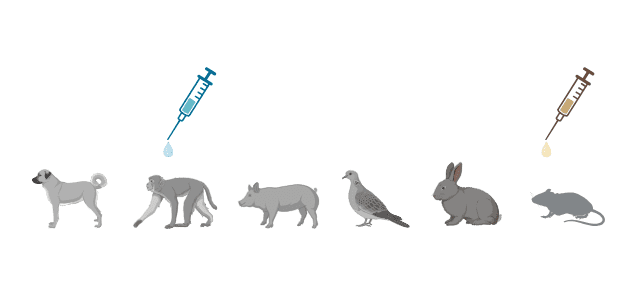
Why Is Animal Testing Problematic?
Animal testing is problematic due to both ethical and scientific shortcomings. Ethically, it involves inflicting physical pain and psychological distress on sentient creatures, raising significant moral concerns about animal suffering and rights. This has led to increasing public opposition and demands for more humane research methods.
Scientifically, animal tests are time-consuming, resource-intensive, and often fail to accurately predict human responses, with most of the drugs that appear safe and effective in animal studies failing in human trials. This high failure rate delays medical progress, wastes resources, and poses risks to clinical trial volunteers. Furthermore, animal models often do not accurately replicate human biology, resulting in unreliable data, whereas more human-relevant research tools can provide better insights and accelerate medical advancements.
Regulatory pressures, such as the European Union’s “3Rs” principle—replacement, reduction, and refinement of animal experiments—further highlight the shift towards developing and using non-animal approaches. In 2020, it is estimated that approximately 8 million animals were used for scientific purposes in the European Union as collected and reported by the European Commission.
Given these concerns, exploring alternatives to animal testing becomes essential to advance ethical and effective scientific research.
Overview of Alternatives to Animal Testing
There are several promising alternatives to animal testing that are transforming the field of research:
- In Vitro Models: These methods involve studying cells, tissues, and organs in a controlled environment outside their natural biological context. By examining cellular responses and mechanisms, researchers can gain valuable insights without using whole animals.
- Computational Models: These models use computer simulations to predict how substances will interact with biological systems. They analyze large datasets quickly and cost-effectively, providing guidance for experimental research and improving our understanding of how different substances might affect human health.
- Organs-on-a-Chip: This cutting-edge technology involves integrating human cells into microfluidic devices that mimic the structure and function of human organs. Organs-on-a-chip replicate the complex interactions within human tissues, offering a more accurate and humane way to study human physiology, diseases, and drug responses.
Ethical and Practical Advantages of Organs-on-a-Chip
The development of organs-on-a-chip technology has not only advanced the precision and scope of biomedical research but also addressed significant ethical concerns related to animal testing. This section explores the foundational principles of organs-on-a-chip technology, how it operates, and how it stands as an ethical and practical advancement in the field of biomedical research.
What is Organs-on-a-Chip Technology?
As just mentioned above, organs-on-a-chip are microengineered devices designed to simulate the functional units of human organs. These chips incorporate living cells within microfluidic environments, replicating the biological activities, mechanics, and physiological responses of entire organs and systems. These systems use advanced biomimicry and microfluidics to create environments that closely mimic the human body’s microarchitecture, providing a powerful tool for studying organ functions and responses, and human biology and disease without relying on animal models.
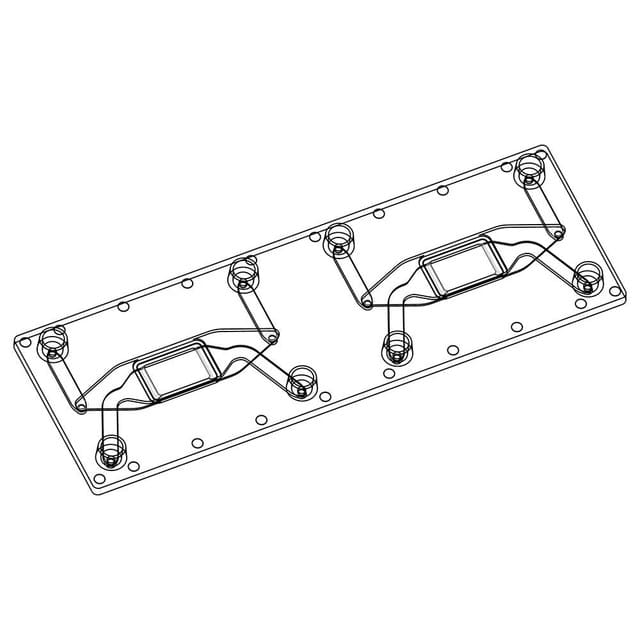
How Does Organs-on-a-Chip Technology Work?
Organs-on-a-chip are small-scale flexible polymer devices, created using advanced microfabrication techniques to develop tiny, cell-cultured platforms that emulate the physical and biological environments of human organs. Typically, these sophisticated systems are composed of several key components:
- Microfluidic Channels: These tiny channels facilitate the controlled flow of fluids, imitating blood flow within human tissues and providing a continuous supply of nutrients and oxygen.
- Biomaterials: Specialized substrates and scaffolds within the chip provide structural support for the growth and organization of human cells. These biomaterials help recreate the extracellular matrix found in natural tissues, promoting realistic cellular behavior and tissue development.
- Human Cells: The chips are seeded with living cells, specific to the organ being simulated, such as lung, liver, or heart cells. These cells are used to create a physiologically relevant model to a particular organ.
- Sensors and Actuators: Integrated sensors continuously monitor conditions such as pH, oxygen levels, and cellular responses in real-time, allowing precise control and measurement of the microenvironment.
The journey of organs-on-a-chip (OoC) technology began in the early 2000s with the creation of the first lung-on-a-chip, designed to emulate the airways of the human lung. The technology saw significant advancements and has since gained widespread adoption by major pharmaceutical companies, academic institutions, and government agencies. This breakthrough in 2010 led to the development of over 15 different organ models, including the intestine, kidney, skin, and blood-brain barrier.
To understand the various types and their unique benefits, here’s a detailed comparison of different organs-on-a-chip models presented in the table below.
Type | Description | Features | Applications |
---|---|---|---|
Lung-on-a-Chip | Simulates the human lung's air-blood barrier. | Human alveolar and endothelial cells separated by a porous membrane; microfluidic channels for airflow and blood flow. | Studying respiratory diseases, drug delivery to lungs, and toxicity testing of airborne substances. |
Heart-on-a-Chip | Mimics the human heart's muscle contractions and electrical activity. | Human cardiac cells aligned on a flexible substrate to replicate rhythmic beating and response to stimuli. | Drug testing for cardiovascular diseases, cardiac toxicity screening, and modeling heart disease. |
Liver-on-a-Chip | Models the human liver's metabolic functions and drug-processing capabilities. | Hepatocytes (liver cells) in a microfluidic environment to replicate biochemical activities. | Drug metabolism studies, liver disease research, and toxicity testing. |
Kidney-on-a-Chip | Replicates the human kidney's filtration and reabsorption processes. | Kidney epithelial cells to model nephrons; microfluidic channels to simulate blood flow. | Drug nephrotoxicity testing, kidney disease research, and studying renal filtration. |
Gut-on-a-Chip | Simulates the human gastrointestinal tract's environment and functions. | Intestinal epithelial cells, immune cells, and sometimes microbiota in a microfluidic setup to replicate peristalsis and nutrient absorption. | Studying gut microbiome interactions, drug absorption, and gastrointestinal diseases. |
Brain-on-a-Chip | Mimics the blood-brain barrier and neural tissue. | Neurons, astrocytes, and endothelial cells to replicate the brain's environment and its interaction with the circulatory system. | Neurological disease research, drug delivery to the brain, and neurotoxicity testing. |
Skin-on-a-Chip | Replicates human skin's barrier and metabolic functions. | Layers of human skin cells to mimic the epidermis and dermis, sometimes incorporating hair follicles or sweat glands. | Studying skin diseases, drug penetration, and cosmetic testing. |
Multi-Organ Chips | Integrates multiple organ models on a single chip to study inter-organ interactions. | Connects different organ chips (e.g., liver, heart, lung) with microfluidic channels to simulate systemic responses. | Comprehensive drug testing, studying disease progression across organs, and personalized medicine. |
Given the significant innovations of organs-on-a-chip technology, an important question arises, especially in the context of comparison with animal testing: Is OoC ethical?
Is Organs-on-a-Chip Ethical?
Organs-on-a-chip technology is considered humane and ethical because it significantly reduces and, in some cases, replaces the need for animal testing. It addresses major ethical concerns associated with the use of animals in research as it eliminates concerns about serious welfare issues including procedures that can cause pain, suffering, and long-term harm to animals.
Additionally, this technology aligns with the principles of the 3Rs—Replacement, Reduction, and Refinement—of the European Union by offering a direct alternative that replaces the need for animals, reduces the number of animals used in research, and refines the methods to enhance animal welfare. Therefore, by providing a more humane and scientifically relevant model for studying human biology and disease, organs-on-a-chip present a highly ethical and innovative approach to biomedical research.
Advantages of Organs-on-a-Chip over Traditional Animal Testing
Organs-on-a-chip represent a groundbreaking advancement in biomedical research, offering several key advantages over traditional animal testing. One of the most significant benefits of organs-on-a-chip is the accuracy and precision of these devices as they can closely mimic human physiology, providing more relevant and reliable data for studying human health and disease compared to animal models.
Another advantage is the precise control and customization that this technology offers. Researchers can precisely manipulate experimental conditions, enhancing reproducibility and reliability of results, which is difficult with animal models due to biological variability.
Speed and high-throughput capabilities are also notable benefits as organs-on-a-chip enable faster experimentation and efficient screening of large numbers of drugs, accelerating research and development of new treatments.
Finally, while the initial setup may be expensive, this technology can reduce long-term research costs by decreasing reliance on animal subjects and the associated expenses of maintaining animal facilities.
This combination of accuracy, control, speed, and cost-effectiveness makes organs-on-a-chip a powerful, ethical alternative to animal testing. More aspects about the advantages of OoC and their comparison to animal testing can be seen in the comparative table below.
Aspect | Animal Testing | Organs-on-a-Chip |
---|---|---|
Visual Representation | ![]() | ![]() |
Accuracy | May not fully replicate human physiology, leading to variability in results. | Mimics human physiology more closely, providing data more relevant to human health. |
Precision | Limited control over experimental conditions and biological variability. | Allows precise control of experimental conditions (e.g., fluid flow, cell environment). |
Speed | Can be time-consuming, with long study durations. | Facilitates faster experimentation and high-throughput screening. |
Cost | High costs related to animal care, housing, and procurement. | Potentially lower costs over time by reducing the need for animal subjects and associated expenses. The initial setup may be expensive. |
Ethical Considerations | Raises significant ethical issues related to animal welfare and moral objections. | Addresses ethical concerns by reducing or eliminating the use of animals, thus minimizing suffering. |
Reproducibility | Variability in results due to genetic and environmental differences among animals. | Provides consistent and reproducible conditions for experiments. |
High-Throughput Capability | Limited by the time and resources required for each animal study. | Enables high-throughput screening of drugs and compounds. |
Complexity | Can model whole-body effects, but with limitations in replicating specific organ functions. | Models specific organ systems, potentially missing interactions between multiple organs. |
Regulatory Acceptance | Well-established, with extensive historical precedent and regulatory frameworks. | Emerging technology with ongoing efforts to gain regulatory acceptance and establish standards. |
Conclusion
Organs-on-a-chip technology marks a significant advancement in biomedical research, offering a more accurate and ethical alternative to animal testing. While it provides precise simulations of human physiology, challenges remain in replicating the complex interactions within the body’s network.
Despite these limitations, advancements in microfabrication and microfluidics are improving these systems to better mimic the dynamic nature of human organs and their interactions. As research progresses, organs-on-a-chip technology promises to further enhance the accuracy, efficiency, and ethical and scientific standards of biomedical research, transforming the future of human health studies.
Stay tuned for more in-depth insights, informative articles, and practical applications in our upcoming posts. Until then, keep exploring the fascinating world of biomedical research and discovery! 🧬✨
📧 If you have any questions or feedback, please feel free to contact us at contact@darwin-microfluidics.com.
References
- Huh, Dongeun, et al. "Reconstituting organ-level lung functions on a chip." Science 328.5986 (2010): 1662-1668. https://doi.org/10.1126/science.1188302
- Fabre, Kristin, et al. "Introduction to a manuscript series on the characterization and use of microphysiological systems (MPS) in pharmaceutical safety and ADME applications." Lab on a Chip 20.6 (2020): 1049-1057. https://doi.org/10.1039/C9LC01168D
- Leung, Chak Ming, et al. "A guide to the organ-on-a-chip." Nat Rev Methods Primers 2, 33 (2022). https://doi.org/10.1038/s43586-022-00118-6
- Singh, Deepanmol, et al. "Journey of organ on a chip technology and its role in future healthcare scenario." Applied Surface Science Advances 9 (2022): 100246. https://doi.org/10.1016/j.apsadv.2022.100246
- Whitesides, George M. "The origins and the future of microfluidics." nature 442.7101 (2006): 368-373. https://doi.org/10.1038/nature05058
- Harrison, D. Jed, et al. "Capillary electrophoresis and sample injection systems integrated on a planar glass chip." Analytical chemistry 64.17 (1992): 1926-1932. https://doi.org/10.1021/ac00041a030
- Raimondi, Ilaria, et al. "Organ-on-a-chip in vitro models of the brain and the blood-brain barrier and their value to study the microbiota-gut-brain axis in neurodegeneration." Frontiers in bioengineering and biotechnology 7 (2020): 435. https://doi.org/10.3389/fbioe.2019.00435
- Ponmozhi, J., et al. "Development of skin-on-a-chip platforms for different utilizations: Factors to be considered." Micromachines 12.3 (2021):294. https://doi.org/10.3390/mi12030294
- Ribas, João, et al. "Cardiovascular organ-on-a-chip platforms for drug discovery and development." Applied in vitro toxicology 2.2 (2016): 82-96. https://doi.org/10.1089/aivt.2016.0002
- Lee, Jeonghwan, and Sejoong Kim. "Kidney-on-a-chip: a new technology for predicting drug efficacy, interactions, and drug-induced nephrotoxicity." Current drug metabolism 19.7 (2018): 577-583. https://doi.org/10.2174/1389200219666180309101844
- Xiang, Yunqing, et al. "Gut-on-chip: Recreating human intestine in vitro." Journal of tissue engineering 11 (2020): 2041731420965318. https://doi.org/10.1177/2041731420965318
- Si, Longlong, et al. "A human-airway-on-a-chip for the rapid identification of candidate antiviral therapeutics and prophylactics." Nature biomedical engineering 5.8 (2021): 815-829. https://doi.org/10.1038/s41551-021-00718-9
- Tostões, Rui M., et al. "Human liver cell spheroids in extended perfusion bioreactor culture for repeated‐dose drug testing." Hepatology 55.4 (2012): 1227-1236. https://doi.org/10.1002/hep.24760
- Zhao, Yi, et al. "Multi-organs-on-chips: towards long-term biomedical investigations." Molecules 24.4 (2019): 675. https://doi.org/10.3390/molecules24040675