The revolution of microfluidics in the world of research, whatever the application, has led to an explosion in the range of materials and processes used to manufacture these devices. Today, the lithography process and its variants appear to be the most obvious in view of the dominance of microfluidic devices manufactured with polymers like PDMS. In this review, we will focus on the main microfabrication processes for polymers in microfluidics.
Photolithography
Photolithography is an inexpensive, simple, fast and reliable manufacturing technique because it allows patterns to be obtained in a consistent manner. This technique uses a mixture of hydrogel precursor and photoinitiator. They then undergo a cross-linking reaction when exposed to ultraviolet (UV) light (Figure 1). The advantage of this technique is that the UV exposure on the hydrogel precursor can be controlled by using a photomask on which the desired patterns are pre-printed [Rivest C et al. 2007 J Mech Mater Struct]. When the photocrosslinking is done, the unreacted polymer is simply washed off. Photolithography allows resolutions of the order of millimeters to micrometers, which offers infinite possibilities for research, especially in tissue engineering.
Moreover, it can be used in combination with other techniques, which makes it very popular. The disadvantages of this technique are the need to use photocurable polymer precursors and the possibility of DNA damage in cells due to the photoinitiator, which forms free radicals when exposed to UV light [Slaughter BV et al. 2009 Adv Mater].
Soft-lithography
Soft-lithography is also a widely used approach for the manufacture of polymers of controlled shape and size (Figure 1).
Soft lithography which includes microcontact printing (μCP), micro-transfer molding (μTM), replica molding (REM), micro molding in capillaries (MIMIC), injection molding, and many other processes [Sahin et al. Fundamental Biomaterials: Metals] can be used in a multitude of applications, such as biosensors, cell adhesion direction, drug screening, cell sorting, and tissue engineering.
The principle of soft lithography is the molding or printing of patterns of biological molecules with well-controlled spatial distribution and topography [2]. This technique is mainly used to produce micro-scale polymers of controlled shape. Specifically, a patterned elastomeric mold is placed on a liquid prepolymer solution, which is then allowed to polymerize, thus providing the complementary characteristics of the hydrogel. During this process, the liquid fills the patterns on the elastomer replica thanks to the phenomena of surface tension and capillary flow.
A number of advantages make this technique widely used, such as ease of access, simplicity, cost-effectiveness, flexibility, versatility, and time efficiency. This technique allows precise control of the size and shape of the structures created [16], and does not require expensive instrumentation [Whitesides GM 2001 Annu Rev Biomed Eng]. However, soft lithography has limitations with ionically or chemically cross-linked hydrogel substrates, such as chitosan or alginate [Franzesi GT et al. 2006 J Am Chem Soc].
PDMS is the material of choice for making micron-sized molds. PDMS is manufactured by mixing a silicon elastomer and a curing agent, which is then poured onto a micro-modeled and thermally cured matrix. This produces the corresponding replica to be used for micro-modeling to produce hydrogel microstructures [Shin H 2007 Biomaterials]. As already mentioned in the materials review, PDMS is a porous biocompatible elastomer, which is preferred in the use of cell cultures. In addition, its surface can be made hydrophilic by the use of a plasma cleaner. In addition to PDMS, polyimides, epoxies, and polyurethanes are also used in molding studies.
Bioprinting
Bioprinting is a technique based on computer-aided design (CAD). In this technique, the deposition of polymers is carried out using a printer in a controlled manner in time and space (Figure 1) [Song SJ 2010 Artif Organs]. CAD-based technologies have recently become popular in the manufacture of porous scaffolding, as has 3D printing. Here, the cells can be introduced into the secondary structures during the printing phase [Khalil S and Sun W 2009 J Biomech Eng]. This technique will enable the generation of complex 3D microenvironments using these bio-printing systems, where cells are embedded in polymers to mimic natural tissues [Song SJ 2010 Artif Organs].
This approach allows high-precision control over the shape and size of features (resolution of tens or hundreds of μm). The most common approaches to bioimprinting include cellular inkjet printing, cellular deposition using a syringe, laser writing [Song SJ 2010 Artif Organs], laser-induced bioimprinting [Guillotin B and Guillemot F 2011 Trend Biotechnol], and rapid prototyping technologies [Buyukhatipoglu K et al. 2010 Tissue Eng Part C-Methods]. Inkjet printing is a fast and cost-effective technique, but has many drawbacks such as mechanical forces on the cells and the fact that the print nozzles can become clogged at high densities [Guillotin B and Guillemot F 2011 Trend Biotechnol].
Laser-assisted bio-printing (LAB), another bio-printing technique, is a useful approach for printing cells, polymers, or biomolecules. This technique uses a laser-absorbing intermediate layer and therefore does not allow direct interaction between the ink and the laser beam [Guillemot et al. 2010. Nanomedicine].
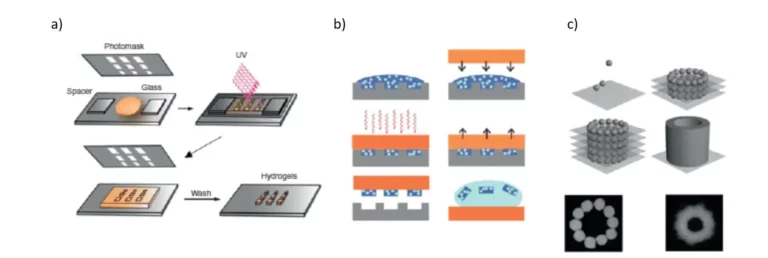
Microfluidic systems
The microfluidic systems use an elastomeric stamp to create channels through a substrate where the ink flows through the capillary phenomenon. By placing the stamp on the substrate, a seal is formed where the stamp is in direct contact with the substrate, preventing the solution from flowing out of the channels.
Microfluidic systems offer several advantages over conventional small-scale hydrogel manufacturing techniques. Microfluidics can produce homogeneous droplets of different sizes. The limitation of these systems is that they are not evolutionary and allow to obtain mainly spherical particles [Franzesi GT et al. 2006 J Am Chem Soc].
It has been reported that continuous hydrogel microfibres with unfit physical and chemical properties have been generated by microfluidic platforms [Kang et al. 2011 Nature Mater]. A microfluidic chip has also been used in combination with a spinning technique to produce multifunctional fibres controlled at the micron scale. These fibres can be created with a different topography or chemistry. They can also contain several types of cells allowing for a variety of clinical applications.
3D Cell Culture: Advantages and Disadvantages
[…] Bioprinting, using bioinks to recreate 3D structures, from scaffolds, to organs. […]