Definition of Digital Microfluidics
Digital microfluidics is a sub-discipline of microfluidics focused on the manipulation of discrete droplets at the microscale. This technology has attracted considerable interest for its potential applications in a wide range of fields, including chemistry, biology, and medicine. The fundamental principle of digital microfluidics is the use of electric fields to control the movement of droplets on a hydrophobic surface, which allows for precise control over fluid volumes and mixtures.
Principles of Digital Microfluidics
The fundamental principle of digital microfluidics is electrowetting, a phenomenon that allows for the manipulation of droplets on a hydrophobic surface using electric fields. By applying a voltage to a droplet, the surface tension can be reduced, allowing the droplet to spread out. This change in surface tension enables precise control over droplet movement, mixing, and splitting, which is essential for various applications in digital microfluidics
Another important principle in digital microfluidics is the use of a dielectric layer, typically composed of materials like silicon dioxide or parylene. This layer insulates the electrodes that generate the electric fields enabling droplet manipulation without direct electrical contact, which can prevent electrolysis and other unwanted reactions.
Electrowetting
Electrowetting involves adjusting the contact angle of a droplet on a hydrophobic surface by applying a voltage. The contact angle, which measures how a liquid interacts with a solid surface, decreases as the applied voltage increases, causing the droplet to spread.
This relation between the contact angle and the applied voltage, described by the Young-Lippmann equation, is fundamental to the movement and control of droplets in digital microfluidics.
Dielectric Layer
The dielectric layer in digital microfluidics is essential for enabling the safe and effective manipulation of droplets without direct electrical contact. Typically made from materials like silicon dioxide or parylene, which have high dielectric constants and excellent insulating properties, this layer insulates the electrodes that generate electric fields.
This insulation prevents unwanted reactions, such as electrolysis, that could interfere with droplet integrity. The thickness and material properties of the dielectric layer are critical design factors, as they directly influence the device’s performance, including voltage control and droplet behavior.
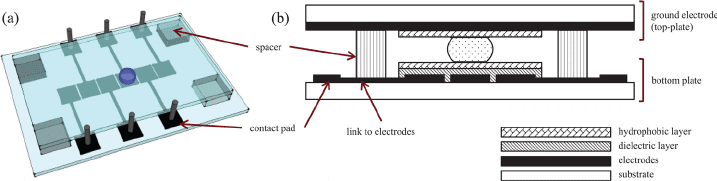
Applications of Digital Microfluidics
Digital microfluidics has a wide range of potential applications, notably in the development of lab-on-a-chip devices for point-of-care diagnostics and high-throughput screening in drug discovery. The ability to precisely control the movement, mixing, and splitting of droplets on a microscale opens up new possibilities for experimentation and application.
Lab-on-a-Chip Devices
Integrating digital microfluidic devices with detection technologies, such as optical or electrochemical sensors, enables the creation of advanced lab-on-a-chip systems capable of performing a wide array of diagnostic tests. The precise manipulation of minute fluid volumes makes digital microfluidics ideal for developing compact, portable diagnostic tools. These lab-on-a-chip devices can conduct complex biochemical analyses, such as the detection of disease biomarkers like those for cancer, providing rapid and accurate diagnostics at the point of care. This integration not only enhances the efficiency of diagnostics but also facilitates timely medical interventions.
High-Throughput Screening
High-throughput screening is a critical method in drug discovery for identifying potential drug candidates by testing a vast array of compounds for their biological activity. Digital microfluidics significantly enhances this process by enabling precise control over fluid volumes and mixtures, allowing for highly efficient screenings. The technology’s ability to operate with minimal sample volumes not only reduces the cost and time of testing but also allows for thousands of parallel assays, vastly accelerating the identification of promising drug candidates.
For instance, digital microfluidic devices have been employed to screen for inhibitors of enzymes like kinase, demonstrating their capacity to streamline and optimize high-throughput screening. This makes digital microfluidics an invaluable tool in modern drug discovery efforts.
Challenges and Future Directions
Despite the significant potential of digital microfluidics, there are still several challenges that need to be addressed. These include issues related to the fabrication and operation of digital microfluidic devices, as well as the integration of these devices with other technologies.
Fabrication Challenges
The fabrication of digital microfluidic devices, which includes the precise deposition of dielectric layers and the patterning of electrodes, is critical to device performance. For example, the thickness and material properties of the dielectric layer can affect the performance of the device, and therefore, are important factors to consider in the design and fabrication process.
Advancements in materials science and microfabrication techniques are anticipated to address these challenges. For instance, utilizing novel dielectric materials with higher dielectric constants and superior insulating properties can enhance device performance. Similarly, improvements in patterning techniques can increase the precision and reliability of electrodes, leading to better control over droplet manipulation and more dependable digital microfluidic devices.
Integration Challenges
Another challenge in digital microfluidics is the integration of these devices with other technologies, such as sensors and microelectronics. For instance, integrating digital microfluidic systems with detection technologies like optical or electrochemical sensors presents difficulties related to compatibility and interfacing.
Ongoing research in this field is expected to overcome these challenges and further expand the potential applications of digital microfluidics. For instance, advances in microfabrication and microelectronics are expected to improve the integration of digital microfluidic devices with other technologies. By integrating these devices with microfabricated sensors and actuators, more complex and versatile devices can be developped, allowing for new applications in areas like point-of-care diagnostics and high-throughput screening.
Conclusion
Digital microfluidics represents a powerful tool for controlling fluid manipulation on a microscale, with significant potential in healthcare, drug discovery, and beyond. While there are challenges to overcome, particularly in fabrication and integration, continued research and development are likely to unlock new possibilities, opening the way for more sophisticated and versatile applications that could transform healthcare and various other fields., which includes the precise deposition of dielectric layers and the patterning of electrodes, is critical to device performance.