Liposomes or lipid nanoparticles are enclosed vesicles widely used for the encapsulation of different kinds of molecules such as DNA, proteins, drugs, and/or other chemicals. In this short review, we will discuss the most used preparation methods with a specific focus on microfluidics.
A Liposome shell is a phospholipid bilayer that acts as a biomimetic membrane enclosing a liquid compartment as schematized in Fig.1. Thanks to their extremely high biocompatibility, liposomes show useful applications in different fields and the development of robust preparation methods is of high importance.
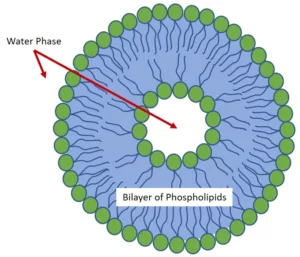
Regardless of the preparation methods, phospholipids are amphiphilic molecules that spontaneously organize in liposomes when in aqueous solutions due to their hydrophobicity. This process reduces the unfavorable interactions between the hydrophobic acyl chains and the surrounding aqueous phase.
According to the specific method used for the preparation of liposomes, the size, the lamellarity and the dispersity of the obtained liposomes can be highly different. The lipid vesicles used in medical applications typically are unilamellar and have a diameter of ~100 nm.
Microfluidic devices for liposome preparation
The oldest and traditional methods for the preparation of liposomes consist of the so-called macroscale or batch techniques based on the initial dehydration of lipid films followed by the swelling process and the final mechanical manipulation to create the water-dispersed bilayers. However, poor reproducibility, limited process control, and the inefficient use of materials and reagents characterize these methods.
Microfluidics has been recently investigated as an efficient method for the preparation of liposomes in a controlled, reproducible, and high-throughput manner. Microfluidics allows a reduction of the used volumes and the associated costs by handling fluids in geometrically constrained compartments typically characterized by micrometer length scales and low Reynolds numbers.
Droplet generator
Microfluidic flow focusing is one of the first introduced methods and consists of the use of microfluidic chips with a cross-flow geometry to ensure the intersection of a central flow of an alcoholic solution containing the phospholipids with an aqueous solution. Typically, three flows merge in the same microfluidic channel as shown in Fig.2. This is the typical pattern of microfluidic devices suitable for the generation of droplets (click here to see some examples). As you can see a phospholipid alcoholic solution is injected in a central channel flanked by two lateral aqueous solutions. The lipid-containing stream is focused, and thanks to 1) the low Reynolds number and 2) the diffusion dominated by the mass transfer, the alcohol diffuses into the aqueous solution and its concentration in the central stream starts to decrease. As it reaches a critical concentration below the solubility of the phospholipids, these ones spontaneously organize into liposomes in a self-assembly manner. The volumetric flow rate ratio between the lipid and water phase streams, together with the total flow rate, influence the size of the obtained liposomes and their adjustment allows a controlled preparation.
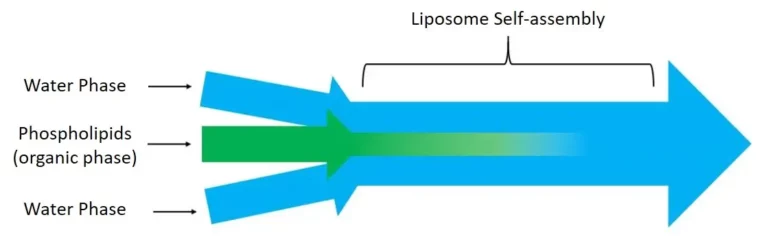
Double emulsion is a variation of the flow-focusing method and consists of the creation of a water-in-oil-in-water emulsion, in which an organic solution of toluene and chloroform is used for the evaporation of the oil phase during the first step. Once the oil phase is removed, the phospholipid monolayers that represent the internal and external oil-water interfaces come in contact with the second aqueous phase and assembly together in liposomes.
This method is technically challenging because it requires the use of two different chips connected in sequence and having hydrophobic coating the first and hydrophilic coating the latter. The efficiency could also be low due to the emulsification step during which the vesicle size and the preparation rate strongly affect 1) their stability and 2) the phospholipid concentration at the oil-water interfaces. Thus, if the liposomes are too small or form too rapidly and the phospholipids are not yet stabilized at the interface, the droplets tend to easily break and release their content, reducing the encapsulation efficiency.
Herringbone mixer
Recently, micromixing through the Herringbone method has been investigated and shows great advantages over the other preparation methods in terms of liposome production efficiency.
For example, PEGylated liposomes were successfully prepared by using a Herringbone Mixer (click here for more information). This device is a chaotic advection mixer able to disrupt the laminar flow typical of microchannels, and reduce the time needed for the liposomes preparation process. The specific pattern of the device made of herringbone grooves redistributes the fluids by creating a transverse flow into the microchannel.
The vertices of the grooves are offset to approximately one third of the width of the channel and two counter-rotating vortices are created by the grooves. The subsequent set of herringbone groves with a mirrored orientation of the vortices allows the creation of a full mixing cycle as shown in Fig.3. The alternation of these herringbone sets reorients the flow periodically and strongly improves the mixing. This microfluidic preparation method allows the controlled creation of liposomes of different sizes (∼100 nm) and good dispersity (< 0.2). By changing the flow rate parameters and the formulations in terms of aqueous buffer, phospholipid concentrations, and composition it is possible to finely tune the production of liposomes with the desired features.
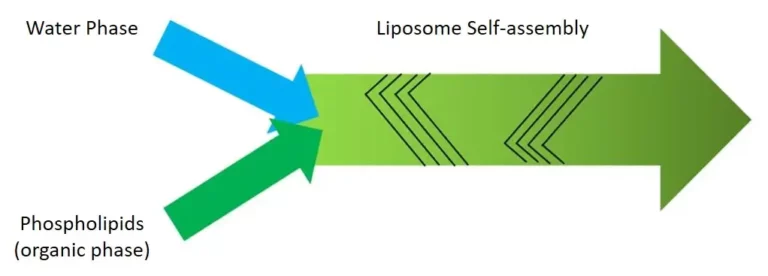
Final considerations
It is clear that microfluidics represents an advantage as method for the preparation of liposomes for many kinds of applications. Compared to the traditional macroscale or batch methods, microfluidics methods allow a high-throughput preparation of liposomes in a well-controlled and reproducible manner. While the progress has been rapid, additional improvements are still needed to establish microfluidics as the method of choice for the preparation of liposomes on both laboratory and industry.
Currently, two are the main obstacles to the affirmation of microfluidics as privileged method for the preparation of liposomes:
- As investigated by Björnmalm et al., (2014), it is difficult for microfluidic un-experienced researchers to have access to microfluidic technologies
- Carugo et al., (2016) and Sackmann et al., (2014), instead, put in evidence the lack of qualified scientific support on how microfluidics could meet their specific needs
Hopefully, the availability of an increasing number of innovative microfluidic devices on the market and the knowledge-sharing attitude of many experienced microfluidicists could now fill this gap and consolidate the position of microfluidics as the preferable method over the conventional ones. Darwin Microfluidics is committed to this revolution. We provide innovative microfluidic devices for liposome preparation and our staff of experienced scientists and PhDs offer the support that researchers need to be successful in their activities.
References
- Björnmalm M. et al., Engineering and evaluating drug delivery particles in microfluidic devices. Journal of Controlled Release 2014, 190: 139.
- Cheung C. C.L. et Al-Jamal W. T. Sterically stabilized liposomes production using staggered herringbone micromixer: Effect of lipid composition and PEG-lipid content. International Journal of Pharmaceutics 2019, 566: 687.
- Carugo D. et al., Liposome production by microfluidics: potential and limiting factors. Scientific Reports 2016, 6: 25876.
- Deshpande S. et Dekker C. On-chip microfluidic production of cell-sized liposomes. Nature protocols 2018, 13 (5): 856.
- Sackmann E.K. et al., The present and future role of microfluidics in biomedical research. Nature 2014, 507: 181.
- van Swaay D. et DeMello A. Microfluidic methods for forming liposomes. Lab on Chip 2013, 13: 752.
- Williams M. S. et al., A practical guide to the staggered herringbone mixer. Lab on chip 2008, 8 (7): 1121.