A widely used microfluidics approach to create emulsions is based on the production of droplets of one fluid dispersed in another immiscible fluid (mainly oils), at frequencies of the order of one to thousands of droplets per second. Droplet-based microfluidics utilizes the interaction between the interfacial tension and the fluidic shear force to break continuous fluids into uniform-sized segments within a microchannel. For this reason, the viscosity of oil can critically impact several parameters such as water-in-oil emulsion, and more precisely how droplet size and droplet generation rate are affected.
With the emergence of microfluidics, the market for oils dedicated to this field has grown considerably. The purpose of this review is to categorise the oils encountered in microfluidics according to their different physico-chemical characteristics and their impact on droplet parameters.
What is a surfactant?
Microfluidics is a process that generates thousands of drops per second, via a chip in which two immiscible fluids circulate in a network of microchannels, forming an emulsion. To stabilise these droplets, it is necessary to add a surfactant. A surfactant is an amphiphilic compound that lowers surface tension. It adsorbs naturally at interfaces because it is composed of a hydrophilic and a hydrophobic part. In this context, it is necessary to find oils compatible with the surfactant and which is compatible with the material used (for example cells).
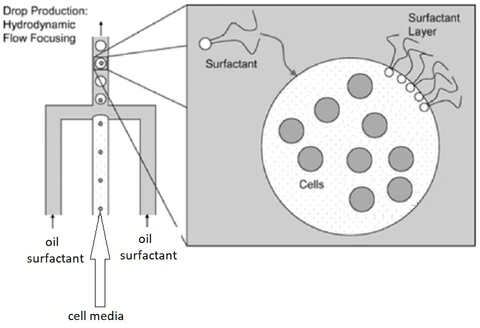
The different types of oils
Typically, mineral oils have been used with cells. For example, focusing on encapsulation mechanisms [Chaber M and Viovy JL. 2008 Proc Natl Acad Sci ; Um E et al. 2010 Apll Phys Lett], and also as a double bacteria emulsification system to screen with incubation times of a few minutes and limited to about 2 hours [Aharoni et al. 2005 Chem Biol]. Among mineral oils, we find hydrocarbon oils. They are therefore limited to the applications such as PCR where the objects of interest (the DNA or RNA fragments) do not exchange between the droplets. Fluorinated oils are the only examples where cell survival and proliferation have been demonstrated with long-term incubation of organisms [Schmitz CHJ et al. 2009 Lab Chip] where gas exchange become crucial. The choice of fluorinated oils is mainly driven by two points : first, they are appealing since most organic compounds are insoluble in these oils. The compounds encapsulated in the droplet should not phase partition and therefore remain in the droplet which solves the exchange limitations of organic and silicone oils. The second advantage of fluorinated oils is their biocompatibility [Baret C. 2012 Lab Chip]. In addition, fluorinated oils are able to solubilise gases, which is critical for cell viability. To a lesser extent, it is possible to use silicone oils but the incompatibility with PDMS means that they remain very little used in microfluidics.Which application for which oil?
In the following tables you will find the oils required for each application and the associated surfactants. These tables have been extracted from Baret 2012 Lab Chip.Silicon oil
Surfactant | DC200 | PDMS | AR20 |
Triton X-100 | PCR (complex oil mix) | ||
SDS (in water) | Oil-in-water emulsification | ||
ABIL EM90 | Directed evolution | ||
No surfactant | Chemical compound storage Emulsification | Raman measurement |
Hydrocarbon oil
Surfactant | Hexadecane | Tetra/octa/dodecane | Mineral oil | Isopar M | Vegetable / organic |
Span 80 | Emulsification Coalescence Droplet splitting Interfacial instabilities Laser manipulation Electrocoalescence Droplet sorting | Emulsification Droplet patterns Chemical coupling | Protein expression Droplet patterns Molecular exchange Cell encapsulation Chemical reactions Electrically-assisted emulsification Electrowetting emulsification | Emulsification Droplet pairing Electrocoalescence | |
Monolein Oleic acid | Tip streaming | Bilayers (squalane) | |||
Tween 20/80 | Dynamic surface tension Emulsification | ||||
Synperonic PEF C12E8 | Dynamic surface tension | Tip streaming | |||
SDS | Dynamic surface tension Emulsification | ||||
n-butanol | Interfacial rheology | ||||
ABIL EM90 | PCR in droplet Directed evolution | ||||
Phospholipid | Lipid bilayer | ||||
No surfactant | Droplet hydrodynamics PCR in droplets | Droplet hydrodynamics (sunflower oil) |
Florinated oil
Surfactant | PFH/PFC/PFD/PFPH | Carrier oil (Raindance Technologies) | HFE/Novec | FC40 | FC70/FC77 | FC3283 |
PF-octanol | Reaction kinetics Protein crystallisation | microPIV | Compound screening Cell sorting | Protein crystallisation | ||
PF-decanol PF-TD acid | PCR in droplet | Protein adsorption | ||||
PF-TD OEG | Protein adsorption Chemistrode | |||||
PFPE- COOH | Splitting droplets Droplet detection | Electrocoalescence | ||||
PFPE- COONH4 | Cells in droplet | Multiple empulsion | Cells in droplets Droplet hydrodynamics Chemical reaction | |||
PFPE-PEG | Cells in droplet PCR Diagnostics | Directed evolution Picoinjector PCR and diagnostics DNA amplification Sorting micro-organims Yeasts in droplets | Cells in droplets Dropspot Coalescence Chemical gradients Droplet separation Electrocoalescence | Molecular exchange Chemical gradients | ||
PFPE-DMP | Cells in droplets Coalescence | Bacteria in droplets | ||||
Short chains | Surface tension and emulsification | |||||
No surfactant | Bacteria/antbiotics | |||||
FluoSurf | Cells in droplet PCR Diagnostics | Water in oil droplet generation Encapsulation Alginate/Polyacrylate/Agarose/Epoxy beads 3D cell culture in droplet PCR and diagnostics DNA Single- cell analysis Cell sorting | Water in oil droplet generation Encapsulation Alginate/Polyacrylate/Agarose/Epoxy beads 3D cell culture in droplet PCR and diagnostics DNA Single- cell analysis Cell sorting |
Compatibility between oils and chip materials
The choice of oil according to the material of the microfluidic device is also a parameter not to be neglected. Indeed, the oil is in close contact with the walls of the channels and a chemical reaction between them could be critical for the expected final result (e.g. formation of droplets of a precise size). Thus, the change of material for the microfluidic system also requires a change in the oil used. Indeed, it is imperative that the oil correctly wets the walls in order to form droplets of water in the oil and not droplets of oil in the water. This short section will therefore allow you to choose the right oil for the materials used in the manufacture of your microfluidic devices.Fluorinated oils
The easiest choice to make remains that of fluorinated oils, since they are compatible with most metals, plastics and elastomers, the best known of which is FC 40. They are also highly recommended in devices made of Teflon/PEEK, especially for droplet nucleation applications in order to get closer to homogeneous nucleation [Ildefonso et al. 2013 J. Am. Chem. Soc.]. In this case, it will be a question of using fluorinated oil of the FMS type.Silicone oils
Of course, silicone oils are highly recommended for microfluidic devices made of silicone. They are also compatible with PDMS or a new material called COC for Cyclic olefin copolymer.Mineral oils
Concerning mineral oils, very little information is available regarding their compatibility with microfluidic device materials. However, it has been reported that these oils can be used well with devices made of polycarbonate (PC).Examples of oil influence on droplet parameters
Impact of oil viscosity on droplet size
In Yao et al. 2019, they used deionized water with red color dye and mineral oil (Zhanhong Chemical Industry, Guangzhou, China) mixed with a surfactant (Span-80, 7.5 g/L, Sigma-Aldrich, St. Louis, MI, USA) as the dispersed and continuous phase, respectively. Four different viscosities (5, 7, 10 and 15 cSt) of the mineral oils were tested to investigate how the different viscosities of the continuous phase affects droplet size and droplet generation rate in water-in-oil emulsion. As can be seen in Figure 2 and table 4 the droplet size decreased as the oil viscosity increased.Pw :Po (mbar) | 5 cSt | 7 cSt | 10 cSt | 15 cSt |
30 :40 60 :80 90 :120 120 :160 150 :200 |
68.3 ± 2.0 43.6 ± 2.1 37.1 ± 1.8 34.0 ± 1.9 32.1 ± 1.0 |
57.0 ± 1.7 39.5 ± 1.8 33.4 ± 2.0 30.7 ± 0.9 29.1 ± 1.1 |
51.0 ± 1.7 35.5 ± 1.7 31.0 ± 1.0 28.1 ± 1.0 26.9 ± 1.1 |
46.3 ± 1.8 32.2 ± 0.9 28.9 ± 0.9 26.0 ± 1.1 25.1 ± 1.3 |
Table 4 : Average diameter of droplets with different oil viscosity and flow pressure (µm) extracted from Yao et al. 2019 Micromachine
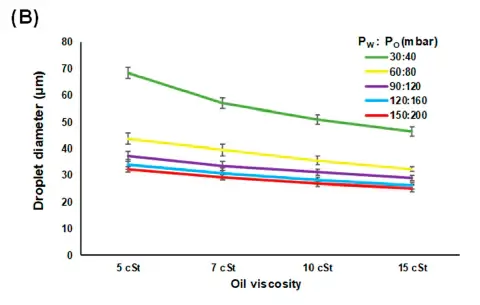
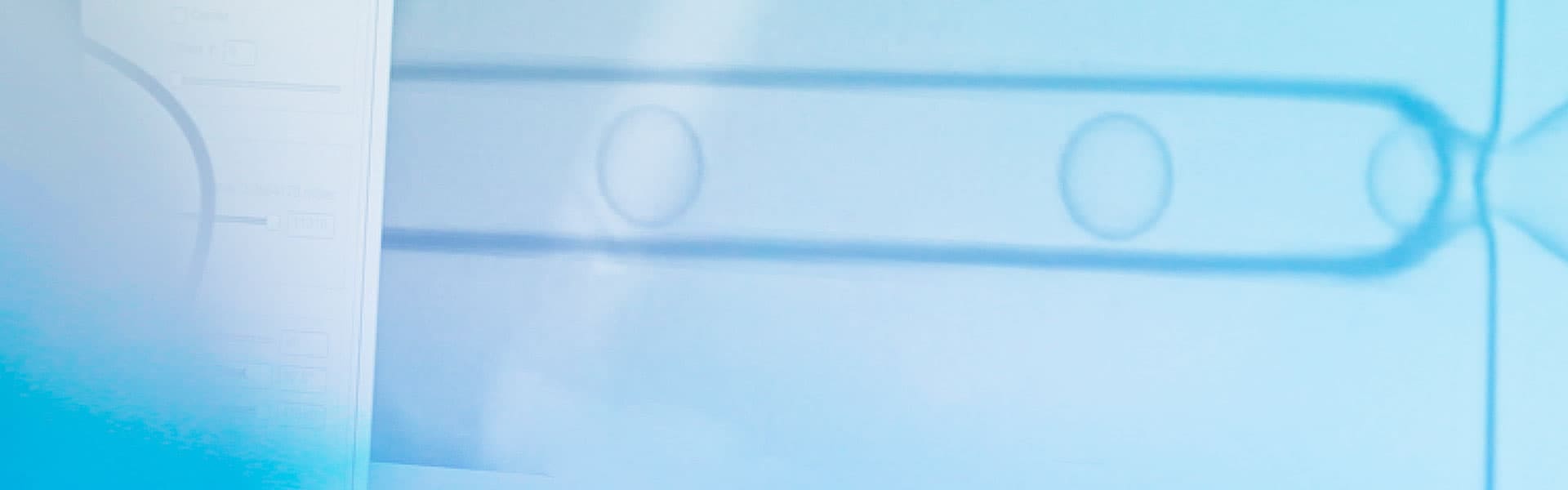
LOOKING TO SCALE UP YOUR DROPLET PRODUCTION?
Explore our scalable solutions designed to meet the demands of high-throughput droplet generation with easeImpact of oil viscosity on droplet generation rate
As shown in table 5 and Figure 3, Yao et al. 2019 shows that the number of droplets generated per unit time decreased as more viscous oil was used under all tested flow pressure conditions, where strong linear correlations were observed (R2 > 0.99 for all flow pressure conditions).Pw :Po (mbar) | 5 cSt | 7 cSt | 10 cSt | 15 cSt |
30 :40 60 :80 90 :120 120 :160 150 :200 |
76 ± 1 157 ± 0 239 ± 8 275 ± 3 581 ± 12 |
66 ± 1 126 ± 2 215 ± 6 334 ± 6 499 ± 12 |
54 ± 1 107 ± 3 182 ± 6 283 ± 3 411 ± 12 |
45 ± 0 93 ± 2 149 ± 1 223 ± 3 305 ± 8 |
Table 4 : Average droplet generation rate with different oil viscosity and flow pressure (µm) extracted from Yao et al. 2019 Micromachine.
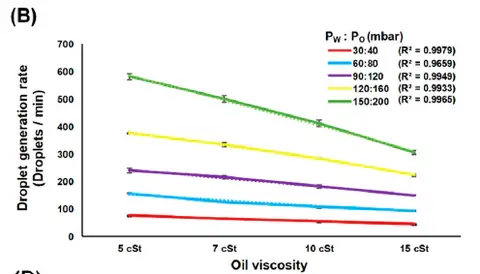
Conclusion
The choice of the oil will essentially depend on your application. However, other parameters must be taken into account such as oil-surfactant and oil-material compatibility. Since the physical parameters of the continuous phase can influence the expected result, it is important to consider, for example, the viscosity of the oil.